EXECUTIVE SUMMARY
Precision medicine is rapidly becoming a reality in clinical practice today. Currently, there are over 10,000 genetic testing products available to clinicians for diagnosing, managing, and treating disease.1 One rapidly growing sector of the genetic testing space is pharmacogenetics, which helps clinicians tailor and optimize medication therapy according to a patient’s genetic profile. Because pharmacogenetics provides information on medication response, rather than disease risk or vulnerability, it has been touted as a more clinically actionable facet of precision medicine.2 The current report aims to provide a comprehensive overview of the evidence landscape for pharmacogenetics in key patient populations.
CENTRAL THEMES THAT EMERGED FROM THE LITERATURE INCLUDE:
1. There is a sizable clinical opportunity for pharmacogenetic intervention in key disease states, including cardiovascular, mental health, and chronic pain therapy.
2. Pharmacogenetic testing requires a paradigm shift for how evidence of clinical utility is generated compared to traditional models of medicine.
3. The clinical evidence supporting validity of testing includes Food and Drug Administration (FDA)-approved labeling for over 100 gene-drug pairs and published expert guidelines for numerous commercially available tests.
4. Such tests may provide the most actionable guidance to clinicians during patient care.
5. The existing clinical utility studies are encouraging and support the potential for pharmacogenetic testing
to improve patient outcomes and reduce health care costs for specific gene-drug pairs.
6. There are a number of important considerations that can facilitate widespread implementation of pharmacogenetic testing in clinical practice.
7. Multiple well-known US medical institutions and centers are paving the way for preemptive (pre- prescription) testing integrated with electronic medical/health records (EMR/EHR) that provide a template for best practices.
INTRODUCTION
Pharmacogenetics (known interchangeably as pharmacogenomics) is the science of how genetics impact an individual’s response to medications. Benefits of pharmacogenetics can range from helping clinicians target medications to improve patient outcomes to enhancing efficiency of pre-market clinical trials for new drug entities.3
Various medication classes such as antidepressants, antipsychotics, analgesics, and oncologics can be ineffective for 20-75% of treated patients.4-7
Opioids | 30-40% |
SSRIs/SNRIs | 38% |
Antipsychotics | 40% |
Asthma drugs | 40% |
Arthritis drugs | 50% |
Alzheimer’s drugs | 70% |
Cancer drugs | 75% |
As clinicians and patients are well aware, optimizing medication therapy can be challenging because response to many commonly used drug classes is often unpredictable, highly variable, and sub-optimal.4-7
Compelling biological and clinical evidence suggests that genetic differences may explain an estimated 20-95% of the variability in medication effects, such as poor response or serious adverse drug reactions (0ADRs).8,9 Testing for clinically relevant genetic variations may offer clinicians an objective tool to help better predict medication response for their patients.10,11 It may also offer significant economic benefits, both in the immediate term by helping clinicians avoid potentially unsafe or ineffective medications2,8; as well as in the long term, since patients on targeted drug treatments are more likely to experience improved health outcomes.12-16
Most commercially available pharmacogenetic tests (PGT) used in clinical practice today are in specialty care, such as oncology, cardiovascular, CNS, and chronic pain therapy.1 As primary care assumes an increasingly prominent role in treating patients with many of these conditions, PGT use is broadening into this clinical setting as well.17 Given the substantial health burden to patients and economic costs associated with these chronic diseases18,19, the potential clinical value of pharmacogenetic testing is significant.20
Over the past three decades, total US health care spending has approximately doubled to 16% of Gross Domestic Product (GDP) and is projected to reach almost 20% of GDP in 2016.21 As both public and private payers struggle with rising health care costs, PGT may provide one solution for improving health care quality and efficiency. For payers, PGT may represent an innovative, science-based tool for targeting use of often- expensive medications to patients most likely to benefit.
Pain and mental health medications were among the top 5 most expensive medications in 2012. Psychiatric, pain, and cardiovascular medications are among the most frequently cited in ADRs.20
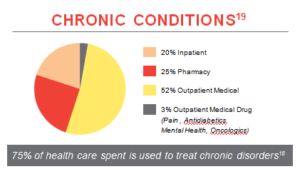
For clinicians, PGT may provide a potentially faster and more effective path to optimizing medication management compared to traditional trial-and-error prescribing. For patients, PGT may offer a relatively simple, non-invasive tool to help them and their care providers maximize the benefits of drug therapy while minimizing the economic, physical, and emotional burdens of ineffective medication trials.
DEFINING THE OPPORTUNITY FOR PHARMACOGENETIC INTERVENTION
Many factors, including diet22, lifestyle23, and age24 contribute to inter-patient variability in medication response. However, genetics remains one of the most compelling targets to evaluate in clinical practice because of the relative ease of measuring and quantifying its impact on patient response. As such, genetic testing may provide a more consistent barometer for predicting medication response among patients compared to other clinical factors and it is also relatively easy to implement in clinical practice.4,17
Nonetheless, it is important to understand that drug pharmacology and clinical response are complex processes that involve multiple biological players. Therefore, it is important to define opportunities for pharmacogenetic intervention, ideally according to clinically relevant gene-drug pairs rather than nonspecific or generic approaches.3 To date, the bulk of the scientific evidence has focused on gene- drug pairs involving metabolism pathways, in particular the CYP450 family of hepatic enzymes.25-29 Genetic variations in CYP450 enzymes may cause a drug to be metabolized either faster or slower than expected for a given patient compared to the reference (or normal) population. Consequently, such patients may have trouble processing and eliminating medications from the body, potentially increasing the risk of therapeutic failure or serious side effects. In general, the consequences may range from unpleasant to fatal depending on the specific gene-drug pair.27
With respect to nomenclature for metabolism genes, the “genotype” denotes the specific DNA changes detected in the enzyme gene, while the “phenotype” describes how DNA variations change the functional activity of the protein encoded by the enzyme gene.
Patients with variant (or non-normal) phenotypes can experience significant differences in their response to certain medications compared to the reference (or normal) phenotype.27
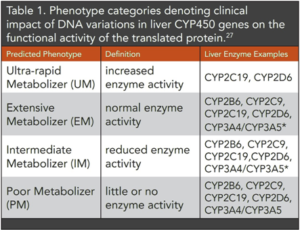
*CYP3A5/CYP3A5: Intermediate Metabolizer displays normal enzyme activity and Extensive Metabolizer displays increased enzyme activity.
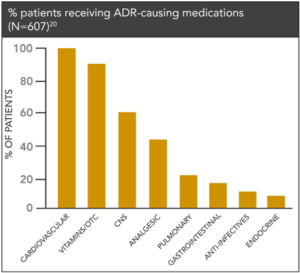
CYP450 enzymes process approximately 90% of medications available in the US and the majority are known to be genetically variable in the general population.30 A systematic 2001 JAMA review reported that CYP450 metabolism pathways are also frequently associated with ADRs and are responsible for metabolizing almost 60% of commonly prescribed medications most frequently cited in adverse events.31 A large (N=607) follow-up study reported that over 1 in 4 primary care patients had received at least one of these “culprit” medications within the previous 12-month period.20
More than 1 in 4 primary care patients take at least one medication associated with ADRs31 and known to be metabolized by genetically variable enzymes.20
For patients receiving cardiovascular, CNS, or analgesic medications, the emergence of ADRs or therapeutic failure can be particularly problematic because they can lead to additional costly treatments, hospitalization, or even death. A large study (N=30,397) of Medicare outpatients found that almost 40% of ADRs reported within a 12-month period were classified as serious, life threatening, or fatal; moreover, CNS, cardiovascular, and analgesics ranked in the top ten ADR-causing medication classes for this population.32
These statistics clearly illustrate the clinical relevance of metabolism pharmacogenetics for certain therapeutic drug classes. They also prompt important questions including:
(i) how common are such genetic variations in the general patient population and (ii) are a significant portion of patients with genetic variations taking medications expected to interact negatively with their genetics. According to a recent retrospective study in a chronic pain cohort (N=102), the vast majority of patients (95%) are variant metabolizers for at least one enzyme pathway that is known to play a primary role in processing medications commonly prescribed in this population (e.g., opioids, benzodiazepines, and antidepressants).33
ALMOST 40% OF ADRS IN MEDICARE PATIENTS WERE CLASSIFIED AS SERIOUS, LIFE THREATENING, OR FATAL WITH CNS, CARDIOLOGY, AND ANALGESIC MEDICATIONS AMONG THE TOP TEN CITED IN ADRS.32
A larger retrospective study (N=1143) in a heterogeneous patient cohort reports similarly high rates of variant metabolizers.34 Most importantly, it also reports that the majority of patients (52.9%) are variant for two enzymes, while 1 in 10 are variant across three clinically relevant CYP450 pathways (CYP2D6, CYP2C19, and CYP2C9).34
MOST PATIENTS ARE VARIANT FOR TWO OR MORE CYP450 ENZYMES AND 1 IN 10 ARE VARIANTS FOR THREE COMMON CYP450S (CYP2D6, CYP2C19, AND CYP2C9).34
ADRs Reported In Medicare Outpatients Over A 12-month Period (N=30,397)32
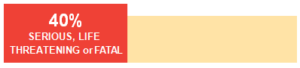
% of patients in general population with variations in CYP450 enzymes (N=1143)34
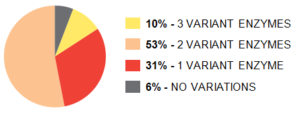
1 IN 3 CASES OF PREDICTED MAJOR DRUG INTERACTIONS IS DUE TO GENETIC VARIATION IN PATIENT METABOLISM.34
These frequency data are especially relevant to clinicians because they suggest that substantially more patients exhibit genetic variations when examined across multiple genes than expected from frequency distributions within a single gene35 and may therefore benefit from PGT. Verbeurgt and colleagues also demonstrate that many of these patients take medications that may negatively interact with their genetics. In fact, genetics were predicted to cause up to 35% of major medication interactions in this patient population.34
Causes of major interactions predicted in heterogeneous patient population (N=1143)34
Causes of major interactions predicted in heterogeneous patient population (N=1143)34
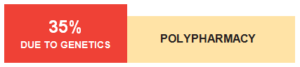
Thus, there is a significant opportunity for PGT in both specialtyand primary care settings and it is only expected to grow in tandem with the accelerating pace of clinical research published daily. Presently, the most robust evidence exists for specific gene-drug pairs that satisfy parameters for being clinically actionable. These gene-drug pairs will be the focus of the current review of the evidence landscap
EXPERT PERSPECTIVES ON EVIDENCE GENERATION
While the potential clinical benefits of PGT are clearly evident, there must be evidence of clinical value to justify routine adoption by clinicians. Payers have traditionally raised the bar even higher for prognostic tests and often require evidence of economic value to justify coverage and reimbursement. To facilitate evidence generation, the Centers for Medicare and Medicaid Services (CMS) have put forth certain criteria that establish a framework for evaluating the analytical, clinical, and economic value of molecular diagnostics used in patient care.36 These criteria provide rough benchmarks that diagnostic developers can use to generate evidence of value; however, there is no clear consensus among stakeholders on the type of studies and/or volume of data that constitute adequate evidence.
Historically, diagnostic laboratories and drug manufacturers have relied on an evidence-based approach that exclusively employs clinical trials that randomize patients into different treatment arms to demonstrate safety and efficacy of the intervention.2,38 Such “gold standard” randomized clinical trials (RCTs) are a staple of traditional medicine and provide effective models to detect the relative benefits and harms of direct interventions, such as drugs or medical devices, in large, representative patient populations. However, there are significant challenges to using the RCT model to validate diagnostics used in precision medicine, such as PGT.2, 37-45 Precision medicine represents a new paradigm of health care that involves matching diagnosis and/or treatment strategies for a patient to their unique molecular makeup. Therefore, the clinical value of PGT is found almost exclusively at the individual patient level.37, 38, 40 Population-based studies often dilute the individual patient signal, and as such, can require sample sizes in the thousands to adequately detect significant genetic associations among treatment groups in RCTs.2 For PGT, this becomes both practically and economically unfeasible given the sheer number of genes and drugs that can be surveyed. Some experts have further reasoned that such evaluation may be clinically overzealous since PGT is not a direct intervention and its use carries negligible harms, especially compared to its potential benefits.2, 37, 40, 42
INCREASINGLY, THE PARADIGM IS SHIFTING AWAY FROM “GOLD STANDARD” TRIALS TO REAL-WORLD, PRAGMATIC STUDIES THAT FOCUS ON PATIENT-LEVEL BENEFITS.119
This has led to a number of research experts2, 42-47 questioning whether genetic testing is being subject to a “higher evidentiary bar”39 compared to non-genetic tests and refer to this bias as “genetic exceptionalism”.37,49 For example, some experts note that renal function (serum creatinine) testing is well-established in clinical practice for guiding drug dosing in renally-impaired patients despite the lack of prospective RCTs that demonstrate evidence of its clinical utility.37, 39, 40, 47 Given PGT’s potential utility and low relative harms, evidence of non-inferiority to current standard of care may be sufficient for clinical implementation, according to some.2 A number of experts and advocates are concerned that the “uneven playing field”47 for evidence requirements for PGT could slow translation of precision medicine into clinical practice.37,39,40,48-53 Some of the difference in standards of evidence between genetic and non-genetic tests may be the familiarity, availability, and perceived low costs of non-genetic tests.2,39,50 However, it is important to note that genetic tests are performed typically once in a patient’s lifetime for a given gene, and this information can be used repeatedly throughout the patient’s life to improve health without incurring additional expense. In contrast, non-genetic tests, such as renal function tests, must be performed and reimbursed a new for each diagnostic incidence in a patient’s lifetime.50 Because of this complexity in its clinical use, some experts have suggested that PGT requires new economic models to accurately assess clinical utility versus current models that may underestimate its clinical impacts during a patient’s lifetime.2,8,50-52
IT IS IMPORTANT TO NOTE THAT GENETIC TESTS ARE PERFORMED TYPICALLY ONCE IN A PATIENT’S LIFETIME FOR A GIVEN GENE, AND THIS INFORMATION CAN BE USED REPEATEDLY THROUGHOUT THE PATIENT’S LIFE TO IMPROVE HEALTH WITHOUT INCURRING ADDITIONAL EXPENSE.
TRADITIONAL MEDICINE VS PRECISION MEDICINE
TRADITIONAL MEDICINE VS PRECISION MEDICINE
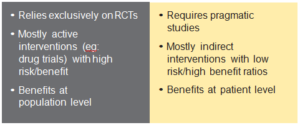
For clinicians weighing the decision to incorporate PGT into patient management, the decision may be ultimately guided by clinical relevance. In the postgenomic era of the last decade, the rate of published pharmacogenetic research has accelerated almost exponentially per year.
Most published evidence to date derives from pragmatic, naturalistic studies conducted in real-world patients,45 which may provide the most valuable assessment of PGT in routineclinical practice according to some experts.37, 38, 40, 42, 51, 53 Nonetheless, it remains challenging for clinicians to distinguish between PGT targets with actionable information that can be implemented immediately in patient care from investigational tests that may presently offer only preliminary value.3,43 However, as the clinical evidence mounts for specific gene-drug pairs, some clinical experts question whether we are shortchanging patient care by waiting for proof-of-value using potentially inadequate models of utility.2, 37, 39, 40, 50
“ARE WE SACRIFICING PATIENT CARE ON THE INSISTENCE OF WAITING FOR PROOF-OF-VALUE VIA THE EVIDENCE-BASED APPROACH?”37
EVIDENCE FOR ANALYTICAL VALIDITY
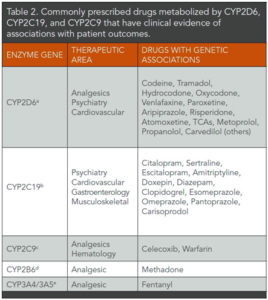
Analytical validity evaluates the accuracy, sensitivity, and reproducibility of the technological platform used to perform PGT.36 As such, this parameter depends on the specific technology platform used to perform the genetic assay.53 The vast majority of commercially available PGTs are designated as laboratory developed test (LDTs). LDTs must meet federally mandated testing standards established by the Clinical Laboratory Improvement Amendments (CLIA) and administered by CMS.These testing standards stipulate criteria for test performance, validity, and quality control that meet specific thresholds for assay specificity, sensitivity, and accuracy.54 A thorough review of PGT technology platforms is beyond the scope of this paper. However, in general, many pharmacogenetic LDTs utilize microarray technology or some variation of polymerase chain reaction (PCR)-based detection. Both technologies are highly accurate and sensitive53, however PCR-based techniques have been in existence for much longer. Microarrays are also limited in the number of genetic variants (alleles) that can be detected per array, while PCR can be easily modified to capture a theoretically unlimited number of variants.53 DNA sequencing technologies represent an emerging PGT platform that is not used widely in the commercial sector as yet.53
EVIDENCE FOR CLINICAL VALIDITY
Clinical validity establishes a positive association between a patient’s genotype and the observed clinical phenotype for specific medications.36 Most of the clinical validity evidence to date has focused on metabolism pathways.25-29,43 These data demonstrate, for example, that a patient’s CYP2D6 genotype accurately predicts their clinical phenotype when prescribed medications primarily processed by the CYP2D6 pathway.
Pharmacogenetic associations between response to the anti-coagulant warfarin (Coumadin®) and variations in two genes, CYP2C9 and VKORC1, were some of the first to be described and currently have the strongest level of clinical evidence.55,56 In 2007, the FDA approved language in the prescribing information to guide clinicians in the use of CYP2C9/VKORC1 genetic information during warfarin administration.57
In recent years, specific algorithms have also been developed that predict precise warfarin maintenance doses based on both genetic and non-genetic factors.58 The platelet inhibitor and prodrug clopidogrel (Plavix®) represents another well-known cardiovascular PGT target. CYP2C19 poor metabolizers produce less active metabolite and are at higher risk of cardiovascular events, especially stent thrombosis.59-62
Antidepressants represent another drug class with well documented pharmacogenetic associations.63 CYP2C19 poor metabolizers taking tricyclic antidepressants (TCAs)64 and SSRIs including sertraline (Zoloft®)65, citalopram (Celexa )66, and escitalopram (Lexapro®)67 may have higher-than-expected plasma concentrations of active medication and are more likely to experience adverse effects. On the other hand, CYP2C19 ultra-rapid metabolizers show lower-than-expected plasma levels of active medication and may be at risk of therapeutic failure.10, 67
Genetic variations for CYP2D6 metabolism also correlate with ADRs and clinical response for many psychiatric medications.10, 26 CYP2D6 poor metabolizers are at higher risk for side effects and discontinuation, while ultrarapid metabolizers are at risk for therapeutic failure with TCAs64, other antidepressants68-70, some antipsychotics71-73, and the ADHD non-stimulant atomoxetine.74 Moreover, a small (N=28) retrospective and naturalistic study found that CYP2D6 poor metabolizers were almost 4-fold over-represented in patients experiencing ADRs due to antidepressants compared to the general population.75 Patients classified as non- responders to their antidepressant therapy were approximately 5-fold over-represented.75
Genetic variations in CYP450 enzymes can also significantly impact clinical outcomes during chronic opioid therapy. The pro-drug codeine is metabolized to its active metabolite morphine via the CYP2D6 pathway. CYP2D6 ultra-rapid metabolizers are at higher risk of toxic morphine accumulation and several cases of respiratory depression and/or death following codeine administration have been reported in children known to be CYP2D6 ultra rapid metabolizers.76-79 A recent population study reports a 16-fold higher risk of CNS depression for nursing infants of mothers that are CYP2D6 ultra-rapid versus poor metabolizers.79
CYP2D6 POOR METABOLIZERS WERE ALMOST 4-FOLD OVER-REPRESENTED IN PATIENTS WHO EXPERIENCED ADRS DUE TO ANTIDEPRESSANTS COMPARED TO THE GENERAL POPULATION. PATIENTS CLASSIFIED AS NON-RESPONDERS TO THEIR ANTIDEPRESSANT THERAPY WERE APPROXIMATELY 5-FOLD OVER-REPRESENTED.75
CYP2D6 variant metabolizers also exhibit poor clinical outcomes for other commonly used opioids80, including tramadol81,82, oxycodone83-85, and hydrocodone.86 CYP2D6 genetic variation may also impact risk for opioid dependence.87
CYP2C19 ultra-rapid metabolizers taking the muscle relaxer carisoprodol (Soma®) are at higher risk of CNS depression, coma, and sometimes death.88-90 CYP2C19 poor metabolizers may experience CNS depression and serotonin syndrome.88-90 CYP2C19 variant metabolizers are also at higher risk of sedation and CNS depression for a number of commonly used benzodiazepines, including diazepam (Valium®).91-94
Finally, fentanyl (Duragesic ) dosing requirements may be impacted by CYP3A4 and CYP3A5 genetic variations95, while CYP2C9 variant metabolizers taking the NSAID pain reliever celecoxib (Celebrex )may be at increased risk of side effects.96-99 Previous studies also demonstrate that CYP2B6 variations are associated with patient drug-taking behavior,149-151 risk of methadone-induced fatality,152 and need for lower methadone doses in patients being treated for addiction.153
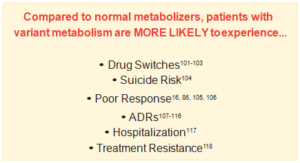
Clinical utility studies evaluate whether PGT improves patient outcomes and associated health care costs.36 Given the inherent limitations of the RCT model,the majority of clinical utility evidence for PGT has employed naturalistic clinical trials in representative patient populations.45 The accumulated data clearly demonstrate that patients with genetically variant metabolism experience a wide variety of poor health outcomes compared to non-variant patients.100-118
Significantly more depressed patients responded and remitted if treatment was guided by PGT results versus standard care.16
Finally, a double-blinded, placebo-controlled RCT (N=33) reported that CYP2D6 poor metabolizers experienced less analgesia with oxycodone than extensive metabolizers in three out of five well-validated pain tests.85 These results also demonstrated that poor metabolizers had statistically lower levels of oxycodone’s more analgesically potent metabolite oxymorphone.85
REAL-WORLD, PRAGMATIC CLINICAL TRIALS
Studies that employ pragmatic designs may include open-label (no comparator group), retrospective, or cross-sectional designs. These studies often have less stringent exclusion criteria and may recruit patients that are more representative of patients treated in routine clinical practice.2, 40, 42 For example, patients on complex polypharmacy or with co-morbid conditions may be frequently excluded from RCTs but recruited into pragmatic trials.
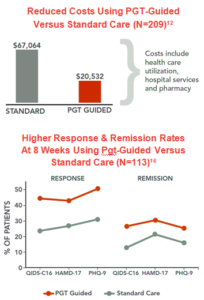
Significantly more depressed patients responded and remitted if treatment was guided by PGT results versus standard care.16
Finally, a double-blinded, placebo-controlled RCT (N=33) reported that CYP2D6 poor metabolizers experienced less analgesia with oxycodone than extensive metabolizers in three out of five well-validated pain tests.85 These results also demonstrated that poor metabolizers had statistically lower levels of oxycodone’s more analgesically potent metabolite oxymorphone.85
REAL-WORLD, PRAGMATIC CLINICAL TRIALS
Studies that employ pragmatic designs may include open-label (no comparator group), retrospective, or cross-sectional designs. These studies often have less stringent exclusion criteria and may recruit patients that are more representative of patients treated in routine clinical practice.2, 40, 42 For example, patients on complex polypharmacy or with co-morbid conditions may be frequently excluded from RCTs but recruited into pragmatic trials.
In depressed patients, naturalistic studies demonstrate that PGT-guided care results in greater therapeutic response and doubled remission rates compared to standard care in the same patient.16,106 In a retrospective study, patients with major depression who were prescribed a medication that was later identified by genetic testing to be problematic had 69% more total health care visits, 67% more general medical visits, 3 times more sick days, and 4 times more disability claims compared to patients who were administered medications considered appropriate for their genetics. These patients also had an estimated greater health care cost of$5,188 per patient.16
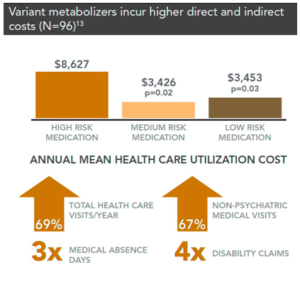
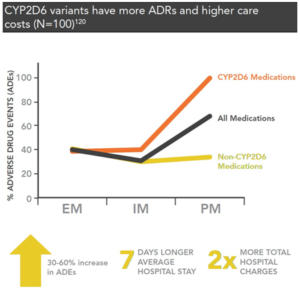
Another open-label, year-long prospective study in psychiatric patients (n=100) receiving in-patient care found that CYP2D6 poor metabolizers are more expensive to treat and have greater ADR risk than extensive (normal) metabolizers’ patients.120 They were also more likely to experience adverse effects with some medications due to reduction in CYP2D6 activity.
MENTAL HEALTH PATIENTS WITH VARIANT METABOLISM USED MORE HEALTH CARE RESOURCES AND INCURRED HIGHER COSTS WHEN PRESCRIBED MEDICATIONS INCOMPATIBLE WITH THEIR GENETICS.13
One potential driver of health care costs in patients with genetic variations is pharmacy costs associatedwith ineffective medications.
PROSPECTIVE 1-YR STUDY FINDS THAT PSYCHIATRIC PATIENTS WITH REDUCED CYP2D6 METABOLISM HAVE SUBSTANTIALLY MORE ADRS AND COST AN EXTRA$4,000–$6,000/YEAR TO TREAT.120
A retrospective study of psychiatric inpatients (N=254) found that the relative risk of medication switches or changes to dosing regimen were significantly greater for CYP2D6 poor metabolizers compared to extensive (normal) patients.101 Another large study (N=1198) in elderly outpatients found that CYP2D6 poor metabolizers receiving TCAs had a higher risk of switching to another antidepressant.102 Poor metabolizers also required a lower maintenance dose of antidepressants compared to extensive (normal) metabolizers.
Compared to normal metabolizers, patients with CYP2D6 poor metabolism (N=254) are101: 4X MORE LIKELY to switch to another antidepressant, 2X MORE LIKELY to change antidepressant dosing, 2X MORE LIEKLY to change antipsychotic dosing.
For many chronic diseases, including depression, hypertension, and diabetes (type 2), lack of medication adherence poses a significant issue that contributes to higher overall medical costs.121-124 In fact, a comprehensive analysis conducted by Medco Health reports that between 50-90% of patients with common health conditions stop taking their prescribed medication by the end of the first year of treatment.125 Multiple studies have shown that medication discontinuation and non-adherence may be driven in part by a lack of medication response and/or medication intolerance.124
Given the association between genetic variations and these drivers of non-adherence, optimizing medication therapy using PGT may improve adherence by reducing the risk of side effects or therapeutic failures. In fact, a number of preliminary studies have shown PGT-guided care may improve adherence rates and clinical outcomes for diabetes therapy121, statins122, and depression therapy.123 A recent large claims database study in depressed patients (N=681) found that PGT-guided treatment significantly improved medication adherenceversus standard care and resulted in a relative total cost savings of almost 10% (or $562) over a 4-month follow- up period.123
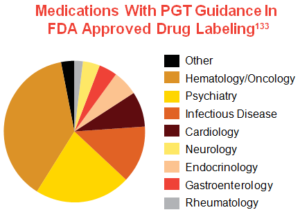
The FDA has approved drug labeling that provides dosing and administration guidance for over 120 medications according to pharmacogenetic information, including carisoprodol, atomoxetine, aripiprazole, celecoxib, clopidogrel, diazepam, codeine, risperidone, paroxetine, tramadol, pantoprazole, and venlafaxine.133 Clopidogrel and codeine include the FDA’s strongest warning (boxed warning) to clinicians about increased risk of side effects or death, respectively, in variant metabolizers.133
OVER 120 MEDICATIONS CARRY PHARMACOGENETIC INFORMATION IN THEIR FDA PRODUCT LABEL133, INCLUDING CODEINE, RISPERDAL®, ABILIFY®, PAXIL®, STRATTERA®, AND ULTRAM®.
CONSIDERATIONS FOR ROUTINE CLINICAL IMPLEMENTATION
Although the evidence for the health and economic benefits of PGT is rapidly accumulating, there remain a number of considerations for successful implementation into routine clinical care.45, 49, 134 One important consideration is clinician awareness and preparedness to interpret and apply genetic test results to manage their patients. According to a number of surveys, the majority of clinicians across a variety of therapeutic specialties have a generally positive perception regarding the potential benefits of pharmacogenetic testing; however, only a small minority of clinicians report feeling adequately informed about this type of testing.135, 136 Many medical organizations and experts are advocating for expanded education on pharmacogenetics in US medical schools and a few industry stakeholders have made efforts to provide non-branded education to clinicians.
Another important stepping stone to routine implementation is achieving consensus among stakeholders regarding the relevant criteria, definitions, and validation models used to assess genetic tests for clinical value.49, 53, 54 Stakeholders must also acknowledge the paradigm shift increasingly advocated by researchers that RCT models are not the only avenue for generating high quality evidence of clinical utility.49 ,53, 54 Integrating PGT information into EMR/EHR systems and sharing patient results across providers is another consideration for routine implementation.46 Despite federal incentives to implement approved EMR systems with interoperable capacity, nationwide adoption of EMR systems that meet these basic criteria has been slow.137 In the absence of EMR/EHR systems, clinicians must be able to appropriately document PGT information within patient health records in ways that facilitate access for patient care.
FUTURE DIRECTIONS
A number of major US medical institutions and centers have implemented PGT into routine care and offer real-world models of best practices for practically translating the clinical value of PGT.138-143 Multiple centers have adopted an innovative approach that couples preemptive (pre-prescribing) PGT with popup alerts embedded within EMR/EHR systems to help guide patient care. Successful examples include preemptive CYP2D6 genotyping at St. Jude’s Hospital138 and the Mayo Clinic141 for psychiatry medications, while University of Florida139, Vanderbilt University140, UNC-Chapel Hill142, and Scripps143 have implemented CYP2C19 testing in coronary intervention patients for Plavix treatment. In fact, Vanderbilt’s PREDICT program has now expanded to include preemptive testing for six genes total (CYP2C19, SLCO1B1, CYP2C9, VKORC1, TPMT, and CYP3A5) and has been implemented during the clinical care of more than 10,000 patients to date. A recent publication of findings from patients enrolled in the PREDICT program suggests that over 91% of patients had one or more actionable variants.144 Importantly, the authors report that when preemptive genotyping is used during patient care, fewer tests are performed compared to reactive testing. Similarly, Mayo’s RIGHT protocol recruited and tested 1,013 participants with the goal of developing best practices for wider implementation of PGT.145 The RIGHT protocol deploys preemptive CYP2D6 testing in addition to sequencing of84 pharmacogenetically relevant genetic variants in a pilot effort to evaluate impacts of EMR-driven pre-prescription PGT during patient care. The RIGHT protocol also aims to circumvent the current clinical paradigm of ordering specific point-of- care PGT upon initiation of treatment, which can be expensive and potentially delay therapeutic decision-making.145
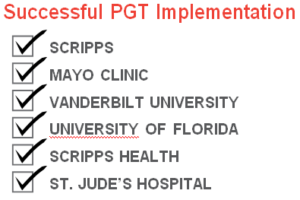
SOME MAJOR MEDICAL INSTITUTIONS AND CENTERS IN THE US ARE TESTING PATIENTS PREEMPTIVELY (PRE-PRESCRIPTION) USING INTEGRATED EHR/EMR SYSTEMS.138-143 IN FACT, VANDERBILT’S PREDICT PROGRAM SUGGESTS THAT PREEMPTIVE TESTING OF PATIENTS PRIOR TO INITIATION OF THERAPY MAY REDUCE TESTING BURDEN COMPARED WITH A REACTIVE POST-PRESCRIPTION STRATEGY.144
Finally, the clinical literature continues to expand for new and emerging genetic targets and these data are likely to improve the precision of pharmacogenetic testing in predicting patient outcomes. These novel biomarkers include genes that encode transport proteins involved in cellular influx and efflux of drugs as well as cell surface receptors that interact with receptors to produce a therapeutic effect.146, 147 Epigenetic biomarkers, which can alter protein expression independent of DNA variations, represent a novel and intensely researched area that may soon be translated from the research arena into clinical practice.148
SUMMARY
In the decade following the decoding of the human genome in 2001, there have been substantial milestones achieved on the path to genomic medicine. Given the potential significance of PGT to patient care and the rapid pace of progress, we must continuously scan the evidence landscape to identify actionable gene-drug pairs for clinical implementation.
In clinical practice today, clear examples of clinically relevant and robustly supported gene-drugs pairs that merit routine implementation during patient management have emerged from the evidence landscape.
“THE POWER IN TAILORED THERAPEUTICS IS FOR US TO SAY MORE CLEARLY TO PAYERS, PROVIDERS, AND PATIENTS: ‘THIS DRUG IS NOT FOR EVERYONE, BUT IT IS FOR YOU.’ THAT IS EXCEEDINGLY POWERFUL.”
-John C. Lechleiter, Ph.D., President & Chief Executive Officer, Eli Lilly & Company42
REFERENCES
1. NextGxDx White Paper. “The genetic testing landscape: Finding the needle in the haystack. A Whitepaper.” Accessed July 21, 2014. http://docs.nextgxdx. com/The%20Genetic%20Testing%20 Landscape%20%20Finding%20a%20 needle%20in%20the%20 Haystack.pdf.
2. Altman, RB. Pharmacogenomics: “Noninferiority” Is Sufficient for
Initial Implementation. Clin Pharmacol Ther. 2011;89(3):348-350.
3. Green, ED, Guyer MS. Charting a course for genomic medicine from base pairs to bedside. Nature. 2011;470:240-213.
4. Spear BB, Heath-Chiozzi M, Huff J. Clinical application of
pharmacogenetics. Trends Mol Med. 2001;7(5):201-204.
5. Ross JS, Ginsburg GS. The Integration of molecular diagnostics with therapeutics;Implications for drug development and pathology practice. Am J Clin Pathol.2003;119:26-36.
6. Katz N, Rauck R, Ahdieh H, et al. A 12-week, randomized placebo- controlled trial assessing the safety and efficacy of oxymorphone extended release for opioid-naive patients with chronic low back pain. Curr Med Res Opin. 2007;23:117–128.
7. Argoff CE. Clinical implications of opioid pharmacogenetics. Clin J Pain. 2010;26(1):S16-20.
8. Crews KR, Hicks JK, Pui C-H, et al. Pharmacogenomics and individualized medicine: Translating science into practice. Clin Pharmacol Ther. 2012;92(4):467-475.
9. Kalow W, Tang BK, Endrenyi L. Hypothesis: comparisons of inter- and intra-individual variations can substitute for twin studies in drug research. Pharmacogenetics. 1998;8:283–9.
10. Samer CF, Lorenzini KI, Rollason V, et al. Applications of CYP450
Testing in the Clinical Setting. Mol Diag Ther. 2013;17(3):165-184.
11. Zhou S-F, Liu J-P, Chowbay B. Polymorphism of human cytochrome P450 enzymes and its clinical impact. Drug Metabolism Rev. 2009;41(2):89-295.
12. Herbild L, Andersen SE, Werge T, et al. Does pharmacogenetic testing for CYP450 2D6 and 2C19 among patients with diagnoses within the schizophrenic spectrum reduce treatment costs? Basic Clin Pharmacol Toxicol. 2013;113(4):266-272.
13. Winner J, Allen JD, Altar CA, et al. Psychiatric pharmacogenomics predicts health resource utilization of outpatients with anxiety and depression. Transl Psychiatry. 2013;3:e242.
14. Hughes, AR, Spreen WR, Mosteller M, et al. Pharmacogenetics of hypersensitivity to abacavir: from PGx hypothesis to confirmation to clinical utility. Pharmacogenomics J. 2008;8, 365–374.
15. Epstein, RS, Moyer TP, Aubert RE, et al. Warfarin genotyping reduces hospitalization rates results from the MM-WES (Medco-Mayo Warfarin Effectiveness study). J. Am. Coll. Cardiol. 2010;55:2804–2812.
16. Hall-Flavin DK, Winner JG, Allen JD, et al. Utility of integrated
pharmacogenomic testing to support the treatment of major depressive disorder in a psychiatric outpatient setting. Pharmacogenet Genomics. 2013;23(10):535-548.
17. Mills R, Voora D, Peyser B, Haga SB. Delivering pharmacogenetic testing in a primary care setting. Pharmacogenomics Personalized Med 2013;6;105-112.
18. Centers for Disease Control and Prevention. Chronic Disease Prevention and Health Promotion. Available online: Accessed July 21, 2014. http://www.cdc. gov/nccdphp/overview.htm.
19. IMS Institute for Health care Informatics. Declining Medicine Use and Costs: For Better or Worse? A Review of the Use of Medicines in the United States in 2012. Accessed July 21, 2014. http://static. correofarmaceutico.com/docs/2013/05/20/ usareport.pdf.
20. Grice GR, Seaton TL, Woodland AM, et al. Defining the opportunity for pharmacogenetic intervention in primary care. Pharmacogenomics. 2006;7(1):61-5.
21. Catlin A, Cowan C, Hartman M, et al. National Health Spending In 2006: A Year Of Change For Prescription Drugs. Health Aff, 2008; 27(1):14-29.
22. Harris RZ, Jang GR, Tsunoda S. Dietary effects on drug metabolism and transport. Clin Pharmacokinet. 2003;42(13):1071-1088.
23. Weathermon R, Crabb DW. Alcohol and medication interactions. Alcohol Res Health. 1999;23(1):40-54.
24. O’Malley K, Crooks J, Duke E, Stevenson IH. Effect of age and sex on human drug metabolism. Br Med J. 1971;3(5775):607-609
25. Altar CA, Hornberger J, Shewade A, et al. Clinical validity of cytochrome P450 metabolism and serotonin gene variants in psychiatric pharmacotherapy. Int Rev Psychiatry. 2013;25(5):509-533.
26. Stingl JC, Brockmöller J, Viviani R. Genetic variability of drug- metabolizing enzymes: the dual impact on psychiatric therapy and regulation of brain function. Mol Psychiatry. 2012;18(3):273-287.
27. Ma Q, Lu AYH. Pharmacogenetics, pharmacogenomics, and individualized medicine. Pharmacol Rev 2011;63(2);437.
28. Xu Y, Johnson A. Opioid Therapy Pharmacogenomics for Noncancer Pain: Efficacy, Adverse Events, and Costs. Pain Res Treat. 2013; ID 943014.
29. Swen JJ, Nijenhuis M, de Boer A, et al. Pharmacogenetics: From Bench to Byte— An Update of Guidelines. Clin Pharmacol Ther. 2011;89(5):662-673.
30. Karczewski KJ, Daneshjou R, Altman RB. Chapter 7: Pharmacogenomics. PLoS Comput Biol. 2012;8(12):e1002817.
31. Phillips KA, Veenstra DL, Oren E, et al. Potential role of pharmacogenomics in reducing adverse drug reactions: a systematic review. JAMA. 2001;286(18):2270-2279.
32. Gurwitz JH, Field TS, Harrold LR, et al. Incidence and preventability of adverse drug events among older persons in the ambulatory setting. JAMA. 2003;289(9):1107-1116.
33. Kirsh KL Ehlenberger E, Huskey A, et al. Exploring rates of abnormal pharmacogenetic findings in a pain practice. J Pain Palliat Care Pharmacother. 2014;28(1):28-32.
34. Verbeurgt, P, Mamiya, T, Oesterheld, J, et al.. How common are drug and gene interactions? Prevalence in a sample of 1143 patients with CYP2C9, CYP2C19 and CYP2D6 genotyping. Pharmacogenomics. 2014;15(5):655–665.
35. Sistonen J, Sajantila A, Lao O, et al. CYP2D6 worldwide genetic variation shows high frequency of altered activity variants and no continental structure. Pharmacogenetics and Genomics 2007;17:93– 101.
36. Palmetto GBA. Molecular Diagnostic Program (MolDXSM): Coverage, coding and pricing standards and requirements. Version 2.0. Accessed May 8,2014 http://www.palmettogba.com/palmetto/moldx. nsf/MolDX_Manual.pdf.
37. Lam YWF. How much evidence is necessary for pharmacogenomic testing implementation? Clin Exp Pharmacol 2012;2:3.
38. Ross S, Anand SS, Joseph P, et al. Promises and challenges of pharmacogenetics: an overview of study design, methodological and statistical issues. J R Soc Med Cardiovasc Dis 2012;1:2
39. Johnson JA. Pharmacogenetics in clinical practice: how far have we come and where are we going? Pharmacogenomics 2013;14(7):835– 843
40. Mrazek, DA, Lerman, C. Facilitating clinical implementation of pharmacogenomics.JAMA. 2011;306(3):304–305.
41. Lam, YWF. Scientific challenges and implementation barriers to translation of pharmacogenomics in clinical practice. ISRN Pharmacol. 2013;Article ID 641089, 17 pages.
42. Personalized Medicine Coalition (PMC) Whitepaper. The case for personalized medicine. 2014, 4th Edition. Accessed July 22, 2014. http://www. personalizedmedicinecoalition.org/Userfiles/
PMCCorporate/file/pmc_the_case_ for_personalized_medicine.pdf.
43. Madian AG, Wheeler HE, Jones RB, et al. Relating human genetic variation to variation in drug responses. Trends Genet. 2012;28(10): 487–495.
44. Moaddeb J, Haga, SB. Pharmacogenetic testing: current evidence of
clinical utility. Ther Adv Drug Saf. 2013; 0(0):1–15.
45. Godman B, Finlayson AE, Cheema PK, et al. Personalizing health care: feasibility and future implications. BMC Medicine 2013;11:179.
46. Pirmohamed M, Hughes DA. Pharmacogenetic tests: the need for a
level playing field. Nature Rev Drug Discovery. 2013;12, 3-4.
47. Roederer MW, Riper MV, Valgus J, et al. Knowledge, attitudes and education of pharmacists regarding pharmacogenetic testing. Personalized Med. 2012;9(1):19—27.
48. Relling MV, Altman RB, Goetz MP, et al. Clinical implementation of pharmacogenomics: overcoming genetic exceptionalism. Lancet Oncol. 2010;11(6):507–509.
49. Dressler LG. Integrating personalized genomic medicine into routine clinical care: Addressing the social and policy issues of pharmacogenomic testing. N C Med J. 2013;74(6):509-513.
50. Sorich MJ, Coory M. Interpreting the clinical utility of a pharmacogenomic marker based on observational association studies. Pharmacogenomics J. 201;14:1-15.
51. Deverka PA, Vernon J, McLeod HL. Economic opportunities and challenges for pharmacogenomics. Annu. Rev. Pharmacol. Toxicol. 2010. 50:423–37.
52. Tunis SR, Stryer DB, Clancy CM. Practical clinical trials: increasing the value of clinical research for decision making in clinical and health policy. JAMA. 2003 290(12):1624–1632.
53. Katsanis SH, Katsanis N. Molecular genetic testing and the future of
clinical genomics. Nat Rev Genetics. 2013;14:415-426.
54. Personalized Medicine Coalition (PMC) Report. “Personalized Medicine Regulation: Pathways for Oversight of Diagnostics”. Accessed December 23, 2013. http://www. personalizedmedicinebulletin.com/wpcontent/uploads/
sites/205/2013/02/ Personalized_Medicine_Regulation-Pathways_for_ Oversight_ of_Dx.pdf
55. Johnson JA, Gong L, Whirl-Carrillo M, et al. CPIC Guidelines for CYP2C9 and VKORC1 Genotypes and Warfarin Dosing. Clin Pharmacol Ther. 2011; 90(4):625-9.
56. Warfarin [Prescribing Information], Princeton, NJ, Bristol-Myers Squibb, 2011. http://www.accessdata.fda.gov/drugsatfda_docs/label/2011/009218s107lbl.pdf
57. FDA News Release. “FDA Approves Updated Warfarin (Coumadin) Prescribing Information New Genetic Information May Help Providers Improve Initial Dosing Estimates of the Anticoagulant for Individual Patients.” Accessed July 22, 2014. http://www.fda.gov/NewsEvents/ Newsroom/PressAnnouncements/2007/ucm108967.htm.
58. Warfarin Dosing website. http://www.warfarindosing.org/Source/ Home.aspx.
59. Bauer T, Bouman HJ, van Werkum JW, et al. Impact of CYP2C19 variant genotypes on clinical efficacy of antiplatelet treatment with clopidogrel: systematic review and meta-analysis. BMJ. 2011;343:d4588.
60. Jang JS, Cho KI, Jin HY, et al. Meta-analysis of cytochrome P450 2C19 polymorphism and risk of adverse clinical outcomes among coronary artery disease patients of different ethnic groups treated with clopidogrel. Am J Cardiol.2012;110(4):502-508.
61. Johnson JA, Roden DM, Lesko LJ, et al. Clopidogrel: a case for indication-specific pharmacogenetics. Clin Pharmacol Ther. 2012;91(5):774-776.
62. Johnson JA, Cavallari LH. Pharmacogenetics and cardiovascular disease– implications for personalized medicine. Pharmacol Rev. 2013;65(3):987-1009.
63. Grasmader K, Verwohlt PL, Rietschel M, et al. Impact of polymorphisms of cytochrome-P450 isoenzymes 2C9, 2C19 and 2D6 on plasma concentrations and clinical effects of antidepressants in a naturalistic clinical setting. Eur J Clin Pharmacol. 2004;60(5):329-336.
64. Hicks JK, Swen JJ, Thorn CF, et al. Clinical pharmacogenetics implementation consortium guideline for CYP2D6 and CYP2C19 genotypes and dosing of tricyclic antidepressants. Clin Pharmacol Ther. 2013;93(5):402-408.
65. Wang JH, Liu ZQ, Wang W, et al. Pharmacokinetics of sertraline in relation to genetic polymorphism of CYP2C19. Clin Pharmacol Ther. Jul 2001;70(1):42-47.
66. Mrazek DA, Biernacka JM, O’Kane DJ, et al. CYP2C19 variation and
citalopram response. Pharmacogenet Genomics. 2011;21(1):1-9.
67. Huezo-Diaz P, Perroud N, Spencer EP, et al. CYP2C19 genotype predicts steady state escitalopram concentration in GENDEP. J Psychopharmacol. 2012;26(3):398-407.
68. Zourkova A, Ceskova E, Hadasova E, et al. Links among paroxetine- induced sexual dysfunctions, gender, and CYP2D6 activity. J Sex Marital Ther. 2007;33(4):343-355.
69. Shams ME, Arneth B, Hiemke C, et al. CYP2D6 polymorphism and clinical effect of the antidepressant venlafaxine. J Clin Pharm Ther. 2006;31(5):493-502.
70. McAlpine DE, O’Kane DJ, Black JL, et al. Cytochrome P450 2D6 genotype variation and venlafaxine dosage. Mayo Clin Proc. 2007;82(9):1065-1068.
71. Llerena A, Berecz R, Penas-Lledo E, et al. Pharmacogenetics of clinical
response to risperidone. Pharmacogenomics. 2013;14(2):177-194.
72. Fleeman N, Dundar Y, Dickson R, et al. Cytochrome P450 testing for prescribing antipsychotics in adults with schizophrenia: systematic review and meta analyses. Pharmacogenomics J. 2011;11(1):1-14.
73. Patsopoulos NA, Ntzani EE, Zintzaras E, Ioannidis JP. CYP2D6 polymorphisms and the risk of tardive dyskinesia in schizophrenia: a meta-analysis. Pharmacogenet Genomics. 2005;15(3):151-158.
74. Michelson D, Read HA, Ruff DD, et al. CYP2D6 and clinical response to atomoxetine in children and adolescents with ADHD. J Am Acad Child Adolesc Psychiatry. 2007;46(2):242-251.
75. Rau, T, Wohlleben, G, Wuttke, H, et al. CYP2D6 genotype: Impact on adverse effects and nonresponse during treatment with antidepressants—A pilot study. Clin Pharmacol Ther 2004;75:386-93.
76. Ciszkowski C, Madadi P, Phillips MS, et al. Codeine, ultrarapid- metabolism genotype, and postoperative death. N Engl J Med. 2009;361(8):827-828.
77. Koren G, Cairns J, Chitayat D, et al.. Pharmacogenetics of morphine poisoning in a breastfed neonate of a codeine-prescribed mother. Lancet. 2006;368(9536):704.
78. Racoosin JA, Roberson DW, Pacanowski MA, et al. New evidence about an old drug-risk with codeine after adenotonsillectomy. N Engl J Med. 2013;368(23):2155-2157.
79. Sistonen J, Madadi P, Ross CJ, et al. Prediction of codeine toxicity in infants and their mothers using a novel combination of maternal genetic markers. Clin Pharmacol Ther. 2012;91(4):692-699.
80. Svetlik S, Hronova K, Bakhouche H, et al. Pharmacogenetics of chronic pain and its treatment. Mediators Inflamm. 2013;ID 864319.
81. Slanar O, Dupal P, Matouskova O, et al. Tramadol efficacy in patients with postoperative pain in relation to CYP2D6 and MDR1 polymorphisms. Bratisl Lek Listy. 2012;113(3):152-155.
82. Gan SH, Ismail R, Wan Adnan WA, et al.. Impact of CYP2D6 genetic polymorphism on tramadol pharmacokinetics and pharmacodynamics. Mol Diagn Ther. 2007;11(3):171-181.
83. Stamer UM, Zhang L, Book M, et al. CYP2D6 genotype dependent oxycodone metabolism in postoperative patients. PLoS One. 2013;8(3):e60239.
84. Zwisler ST, Enggaard TP, Mikkelsen S, et al. Impact of the CYP2D6 genotype on post-operative intravenous oxycodone analgesia. Acta Anaesthesiol Scand. 2010;54(2):232-240.
85. Zwisler ST, Enggaard TP, Noehr-Jensen L, et al. The hypoalgesic effect of oxycodone in human experimental pain models in relation to the CYP2D6 oxidation polymorphism. Basic Clin Pharmacol Toxicol. 2009;104(4):335-344.
86. Stauble, ME, Moore, AW, Langman, LJ, et al. Hydrocodone in postoperative personalized pain management: pro-drug or drug? Clin Chim Acta. 2014;429:26-9.
87. Xu Y, Johnson A. Opioid therapy pharmacogenomics for noncancer pain: Efficacy, adverse events, and costs. Pain Res Treat. 2013;2013:943014.
88. Soma [Prescribing Information], Somerset, NJ. Meda Pharmaceuticals Inc. 2013. http://www.soma250.com/pdf/full_prescribing_info.pdf.
89. Bramness JG, Skurtveit S, Mørland J. Impairment due to intake of
carisoprodol. Drug Alcohol Depend. 2004;74(3):311-8.
90. Bramness JG, Mørland J, Sørlid HK, et al. Carisoprodol intoxications
and serotonergic features. Clin Toxicol (Phila). 2005;43(1):39-45.
91. Inomata S, Nagashima A, Itagaki F, et al. CYP2C19 genotype affects diazepam pharmacokinetics and emergence from general anesthesia. Clin Pharmacol Ther 2005;78(6):647-55.
92. Zhang YA, Reviriego J, Lou YQ, et al. Diazepam metabolism in native Chinese poor and extensive hydroxylators of S-mephenytoin: interethnic differences in comparison with white subjects. Clin Pharmacol Ther. 1990;48(5):496-502.
93. Qin XP, Xie HG, Wang W, et al. Effect of the gene dosage of CgammaP2C19 ondiazepam metabolism in Chinese subjects. Clin Pharmacol Ther. 1999;66(6):642-6.
94. Valium [Prescribing Information], Nutley, NJ, Roche Laboratories, Inc,
2008.
95. Barratt DT, Bandak B, Klepstad P, et al. Genetic, pathological and physiological determinants of transdermal fentanyl pharmacokinetics in 620 cancer patients of the EPOS study. Pharmacogenet Genomics. 2014;24(4):185-94.
96. Chan AT, Zauber AG, Hsu M, et al. Cytochrome P450 2C9 variants influence response to celecoxib for prevention of colorectal adenoma. Gastroenterology. 2009;136(7):2127-2136.e1.
97. Pilotto A, Seripa D, Franceschi M, et al. Genetic susceptibility to nonsteroidal anti-inflammatory drug-related gastroduodenal bleeding: role of cytochrome P450 2C9 polymorphisms. Gastroenterology. 2007;133(2):465-71.
98. Carbonell N, Verstuyft C, Massard J, et al. CYP2C9*3 Loss-of-function
allele is associated with acute upper gastrointestinal bleeding related to the use of NSAIDs other than aspirin. Clin Pharmacol Ther. 2010;87(6):693-8.
99. Malhi H, Atac B, Daly AK, et al. Warfarin and celecoxib interaction in the setting of cytochrome P450 (CYP2C9) polymorphism with bleeding complication. Postgrad Med J. 2004;80(940):107-9.
100. Prows CA, Nick TG, Saldana SN, et al. Drug-metabolizing enzyme genotypes and aggressive behavior treatment response in hospitalized pediatric psychiatric patients. J Child Adolesc Psychopharmacol. 2009;19(4):385-394.
101. Mulder H et al. The association between cytochrome P450 2D6 genotype and prescription patterns of antipsychotic and antidepressant drugs in hospitalized psychiatric patients: A
retrospective follow-up study. J Clin Psychopharmacol.2005;25(2).
102. Bijl MJ, Visser LE, Hofman A, et al. Influence of the CYP2D6*4 polymorphism on dose, switching and discontinuation of antidepressants. Br J Clin Pharmacol.2008;65(4):558-64.
103. Gregoor JG, van der Weide K, van der Weide J, et al. The association between CYP2D6 genotype and switching antipsychotic medication to clozapine. Eur J Clin Pharmacol. 2013;69(11):1927-32.
104. Peñas-Lledó EM, Dorado P, Agüera Z, et al. High risk of lifetime history of suicide attempts among CYP2D6 ultrarapid metabolizers with eating disorders. Mol Psychiatry. 2011;16(7):691-2.
105. Rundell JR, Harmandayan M, Staab JP. Pharmacogenomic testing and outcome among depressed patients in a tertiary care outpatient psychiatric consultation practice. Transl Psychiatry 2011;1, e6.
106. Hall-Flavin DK, Winner JG, Allen JD, et al. Using a pharmacogenomic algorithm to guide the treatment of depression. Transl Psychiatry. 2012;2:e172.
107. Chen S, Wen-Hwei C, Blouin RA, et al. The cytochrome P450 2D6 (CYP2D6) enzyme polymorphism: Screening costs and influence on clinical outcomes in psychiatry. Clin Pharmacol Ther 1996;60:522-34.
108. de Leon J, Susce MT, Pan RM, et al. The CYP2D6 poor metabolizer phenotype may be associated with risperidone adverse drug reactions and discontinuation. J Clin Psychiatry. 2005;66(1):15-27.
109. Jannetto PJ, et al. Utilization of pharmacogenomics and therapeutic drug monitoring for opioid pain management. Pharmacogenomics. 2009;10(7):1157-67.
110. Wuttke H, Rau T, Heide R, et al. Increased frequency of cytochrome P450 2D6 poor metabolizers among patients with metoprolol- associated adverse effects. Clin Pharmacol Ther 2002;72(4):429-37.
111. Gan SH, Ismail R, Wan Adnan WA, et al. Impact of CYP2D6 genetic polymorphism on tramadol pharmacokinetics and pharmacodynamics. Mol Diagn Ther. 2007;11(3):171-81.
112. Scordo MG, Spina E, Romeo P, et al. CYP2D6 genotype and antipsychotic-induced extrapyramidal side effects in schizophrenic patients. Eur J Clin Pharmacol. 2000;56(9-10):679-83.
113. Kobylecki CJ, Jakobsen KD, Hansen T, et al. CYP2D6 genotype predicts antipsychotic side effects in schizophrenia inpatients: a retrospective matched case-control study. Neuropsychobiology. 2009;59(4):222-6.
114. Sutter ME, Gaedigk A, Albertson TE, et al. Polymorphisms in CYP2D6 may predict methamphetamine related heart failure. Clin Toxicol (Phila). 2013;51(7):540-4.
115. Vandel P, Haffen E, Vandel S, et al. Drug extrapyramidal side effects. CYP2D6 genotypes and phenotypes. Eur J Clin Pharmacol. 1999;55(9):659-65.
116. Koski A, Ojanperä I, Sistonen J, et al. A fatal doxepin poisoning associated with a defective CYP2D6 genotype. Am J Forensic Med Pathol. 2007;28(3):259-61.
117. de Leon J, Barnhill J, Rogers T, et al. Pilot study of the cytochrome P450-2D6 genotype in a psychiatric state hospital. Am J Psychiatry. 1998;155(9):1278-80.
118. Zabrocka M, Woszczek G, Borowiec M, et al. CYP2D6 gene polymorphism in psychiatric patients resistant to standard pharmacotherapy. Psychiatr Pol. 1999;33(1):91-100.
119. PubMed search. Citations (http://www.ncbi.nlm.nih.gov/pubmed) searched using keyword “pharmacogenetics” and “cancer” and filtered by publication date
120. Chou WH, et al. Extension of a Pilot Study: Impact From the Cytochrome P450 2D6 Polymorphism on Outcome and Costs Associated With Severe Mental Illness. J Clin Pyschopharmacol. 2000;20(2):246-251.
121. Grant RW, Hivert M, Pandiscio JC, Florez JC, Nathan DM, Meigs JB. The clinical application of genetic testing in type 2 diabetes: a patient and physician survey. Diabetologia 2009; 52: 2299–2305.
122. Charland SL, Agatep BC, Epstein RS, Frueh FW, Herrera V, Devlin J et al. Patient knowledge of pharmacogenetic information improves adherence to statin therapy: results of the additional Kif6 risk offers better adherence to statins (Akrobats) trial. J Am Coll Cardiol 2012; 59: E1848–E1848.
123. Fagerness, J, Fonseca, E, Hess, GP, et al. Pharmacogenetic-guided psychiatric intervention associated with increased adherence and cost savings. Am J Manag Care. 2014;20(5):e146-e156
124. Haga, SB, LaPointe, NMA. The potential impact of pharmacogenetic testing on medication adherence. Pharmacogenomics J. 2013;1-3. doi:10.1038/tpj.2013.33
125. Medco analysis. Health care Reform: The future is now. Accessed July 30,2014. http://www.goldlabcolorado.com/2010/David%20Snow%20 slides.pdf.
126. Relling MV et al. CPIC: Clinical Pharmacogenetics Implementation Consortium of the Pharmacogenomics Research Network. Clin Pharmacol Ther. 2011;89:464-7.
127. Scott SA, Sangkuhl K, Stein CM, et al. Clinical pharmacogenetics implementation consortium guidelines for CYP2C19 genotype and clopidogrel therapy: 2013 update. Clin Pharmacol Ther. Sep 2013;94(3):317-323.
128. Crews KR, Gaedigk A, Dunnenberger HM, et al. Clinical Pharmacogenetics Implementation Consortium (CPIC) guidelines for codeine therapy in the context of cytochrome P450 2D6 (CYP2D6) genotype. Clin Pharmacol Ther. Feb 2012;91(2):321-326.
129. CPIC Dosing Guideline for codeine and CYP2D6. PharmGKB. Accessed July 7, 2014. https://www.pharmgkb.org/guideline/ PA166104996
130. Gharani N, Keller MA, Stack CB, et al. The Coriell personalized medicine collaborative pharmacogenomics appraisal, evidence scoring and interpretation system. Genome Med. Oct 18 2013;5(10):93.
131. Swen JJ, Wilting I, de Goede AL, et al,.Pharmacogenetics: from bench to byte. Clin Pharmaol Ther. 2008;83(5):781-7.
132. Swen JJ, Nijenhuis M, de Boer A, et al. Pharmacogenetics: from bench to byte- an update of guidelines. Clin Pharmacol Ther. 2011;89(5):662-73.
133. US Food and Drug Administration. Table of Pharmacogenomic Biomarkers in Drug Labels. Accessed September 4, 2013. http://www. fda.gov/drugs/scienceresearch/researchareas/pharmacogenetics/ ucm083378.htm
134. Shuldiner, AR, Relling, MV, Peterson, JF, et al. The pharmacogenomics research network translational pharmacogenetics program: Overcoming challenges of real-world implementation.Clin Pharmacol Ther 2013;94(2):207-210.
135. Jürgens G, Jacobsen CB, Rasmussen HB, et al. Utility and adoption of CYP2D6 and CYP2C19 genotyping and its translation into psychiatric clinical practice. Acta Psychiatr Scand. 2012;125(3):228-37.
136. Stanek EJ, Sanders CL, Taber KA, et al. Adoption of pharmacogenomic testing by US physicians: results of a nationwide survey. Clin Pharmacol Ther. 2012;91(3):450-8.
137. Hsiao CJ, Hing E. Use and characteristics of electronic health record
systems among office-based physician practices: United States,
2001-2012. NCHS Data Brief. 2012;(111)1-8. Accessed September 21,
2013. http://www.cdc.gov/ nchs/ data/databriefs/db111.pdf.
138. Crews KR, Cross SJ, McCormick JN, et al. Development and implementation of a pharmacist-managed clinical pharmacogenetics service. Am J Health Syst Pharm 2011;68: 143-150.
139. Nelson DR, Conlon M, Baralt C, et al. University of Florida Clinical and Translational Science Institute: transformation and translation in personalized medicine. Clin Transl Sci 2011;4:400-402.
140. Pulley JM, Denny JC, Peterson JF, et al. Operational implementation of prospective genotyping for personalized medicine: The design of the Vanderbilt PREDICT project. Clin Pharmacol Ther 2012;92: 87-95.
141. Mrazek DA. Psychiatric pharmacogenomic testing in clinical practice. Dialogues Clin. Neurosci. 12(1), 69–76 (2010).
142. Johnson JA, Burkley BM, Langaee TY, et al. Implementing personalized medicine: Development of a cost-effective custom pharmacogenetics genotyping array. Clin. Pharmacol. Ther. 2012;92(4), 437–439.
143. Johnson JA, Cavallari LH. Pharmacogenetics and cardiovascular disease – implications for personalized medicine.Pharmacol Rev. 2013;65:987-1009.
144. Van Driest, SL, Shi, Y, Bowton, EA, et al. Clinically actionable genotypes among 10,000 patients with pre-emptive pharmacogenomic testing. Clin Pharmacol Ther. 2014; doi:10.1038/ clpt.2013.229.
145. Bielinski SJ, Olson JE, Pathak J, et al. Preemptive genotyping for personalized medicine: design of the right drug, right dose, right time-using genomic data to individualize treatment protocol. Mayo Clin Proc. 2014;89(1):25-33.
146. Reynolds GP, McGowan OO, Dalton CF. Pharmacogenomics in psychiatry: the relevance of receptor and transporter polymorphisms. Br J Clin Pharmacol. 2014;77(4):654-72.
147. Sadee W. Gene-gene-environment interactions between drugs, transporters, receptors, and metabolizing enzymes: Statins, SLCO1B1, and CYP3A4 as an example. J Pharm Sci. 2013;102(9):2924-9.
148. Kim IW, Han N, Burckart GJ, Oh JM. Epigenetic changes in gene expression for drug-metabolizing enzymes and transporters. Pharmacotherapy. 2014;34(2):140-50.
149. Lee AM, Jepson C, Hoffmann E, et al. CYP2B6 genotype alters abstinence rates in a bupropion smoking cessation trial. Biol Psychiatry 2007;62:635-41.
150. Quaak M, Van Schayck C, Knaapen A, et al. Genetic variation as
a predictor of smoking cessation success. A promising preventive and intervention tool for chronic respiratory diseases? Eur Respir J 2009;33:468-80.
151. Zhu A, Cox L, Nollen N, et al. CYP2B6 and bupropion’s smoking- cessation pharmacology: the role of hydroxybupropion. Clin Pharmacol Ther 2012;92:771-77.
152. Bunten H, Liang W, Pounder D, et al. CYP2B6 and OPRM1 gene variations predict methadone-related deaths. Addict Biol 2010;16:142-44.
153. Levran O, Peles E, Hamon S, et al. CYP2B6 SNPs are associated with methadone does required for effective treatment of opioid addiction. Addict Biol 2013;18:709-16.
154. Liao Z, Feng S, Ling P, et al. Meta-analysis of randomized controlled trials reveals an improved clinical outcome of using genotype plus clinical algorithm for warfarin dosing. J Thromb Thrombolysis. 2014 Jun 25.
155. Shaw K, Amstutz U, Hildebrand C, et al. VKORC1 and CYP2C9 genotypes are predictors of warfarin-related outcomes in children. Pediatr Blood Cancer. 2014 Jun;61(6):1055-62.
156. Pirmohamed M, Burnside G, Eriksson N, et al. A randomized trial of genotypeguided dosing of warfarin. N Engl J Med. 2013 Dec 12;369(24):2294-303.
157. Anderson JL, Horne BD, Stevens SM, et al. A randomized and clinical effectiveness trial comparing two pharmacogenetic algorithms and standard care for individualizing warfarin dosing (CoumaGen-II). Circulation. 2012 Apr 24; 125(16):1997-2005.
158. Epstein RS, Moyer TP, Aubert RE, et al. Warfarin genotyping reduces hospitalization rates results from the MM-WES (Medco-Mayo Warfarin Effectiveness study). J Am Coll Cardiol. 2010 Jun 22;55(25):2804-12.